Step 1 of 8
Fetal and transitional circulation
Embryology
The main steps of embryologic heart development take place between the 3rd and 8th week post-conception. The primitive heart tube is a mesodermal structure that begins to beat on the 22th day. It includes several parts (Figure 14.1) [7].
- The bulbus cordis, whose distal part (truncus) will form the outflow tracts of the ventricles and the proximal part (conus) of the right ventricle;
- The primitive ventricle will essentially be the future left ventricle;
- The primitive atrium will form the two left and right atria;
- The right horn of the sinus venosus will participate in the RA and the left horn will become the coronary sinus.
Figure 14.1: Embryology of the heart. A: heart tube after 3 weeks; the truncus and the conus together form the bulbus cordis. B: looping of the tube over itself by a rotation bringing the RV around the LV and the primitive atrium upwards and towards the rear. The conus will form the non-trabeculated part of the RV. C: after 28 days, the atrium is at the back and divided into two, the LVG is clearly identified. The LV comprises 2 parts: the conus will be the non-trabeculated part, the trabeculated part is the result of the primitive ventricle; the truncus will form the outflow tracts [6].
This tube will fold in on itself so that the conus is found next to and in front of the primitive ventricle, and the atria behind the ventricles. This movement explains how the RV is wound by growing around the LV (Figure 14.2). The septation of the cavities takes place by the fusion of the upper and lower cushions of the interauricular and intervenricular septum; it is completed by the 6th week. The septation of the truncus is done by a membrane which is built in a spiral, so that the aorta and pulmonary artery wrap around each other when they are completely separated (8th week). The heart valves are completed later: in the 10th week for the ventriculo-arterial valves and in the 12th week for the AV valves [6]. The heart is fully formed on the 50th day.
This tube will fold in on itself so that the conus is found next to and in front of the primitive ventricle, and the atria behind the ventricles. This movement explains how the RV is wound by growing around the LV (Figure 14.2). The septation of the cavities takes place by the fusion of the upper and lower cushions of the interauricular and intervenricular septum; it is completed by the 6th week. The septation of the truncus is done by a membrane which is built in a spiral, so that the aorta and pulmonary artery wrap around each other when they are completely separated (8th week). The heart valves are completed later: in the 10th week for the ventriculo-arterial valves and in the 12th week for the AV valves [6]. The heart is fully formed on the 50th day.
Figure 14.2: A and B: the RV looks like a crescent wrapped around the LV (grey and dotted line). It comprises an admission chamber, a body (with the apex) and a infundibulum with the outflow tract (RVOT) which is tubular shaped. TV: tricuspid valve. PA: pulmonary artery. C: The crista supraventricularis (CS) separates the trabeculated area (admission, apex and body of the ventricle) from the outflow tract. The walls of the RV observed in transgastric short-axis view are the inferior, lateral and anterior walls. This complex form of the RV makes it difficult to measure its volume and its ejection fraction.
Foetal circulation
In utero, blood oxygenation takes place in the placenta and not in the lungs. Blood saturated with O2 (PO2 35 mmHg, SO2 75%) returns to the heart by the umbilical vein and the liver, where it bypasses the portal system by the ductus venosus (DV) or canal of Arantius, which reroutes it directly to the inferior vena cava (IVC) (Figure 14.3). Having entered the right atrium (RA), it is mostly directed to the foramen ovale (FO) by the Eustachian membrane, where it reaches the left atrium (LA). Highly desaturated blood (SO2 40%) of the superior vena cava (SVC) remains in the right heart and reaches the pulmonary artery (PA, SO2 50%). The blood of the PA is directed to the descending aorta by the ductus arteriosus (DA), because pulmonary vascular resistances are very high; flow through the lungs only represents 7% of cardiac output. Thus, blood rich in oxygen and glucose returning from the placenta preferentially perfuses the brain, the coronaries and upper limbs (SO2 65%), whereas the splanchnic organs are supplied by less saturated blood (SO2 55-60%) from the ductus arteriosus [4]. The RV contributes to 55% of the total cardiac output and the LV to 45% [7]. Two-thirds of the flow of the descending aorta goes to the placenta. Systemic circulation and pulmonary circulation operate in parallel.
Figure 14.3: Diagram of foetal circulation. Blood saturated with O2 (PaO2 35 mmHg, SO2 75%) returns to the heart by the umbilical vein and the liver, where it is shunted directly to the IVC by the ductus venosus (DV). Having entered the RA, it is mostly directed to the foramen ovale (FO) by the Eustachian membrane (EM), where it reaches the LA. Highly desaturated blood (SO2 40%) of the SVC remains in the right heart and reaches the pulmonary artery (PA, SO2 50%). The blood of the PA is directed to the descending aorta by the ductus arteriosus (DA), because pulmonary vascular resistances (PVR) are very high. PV: pulmonary veins.
Transitional circulation
At birth, circulation has to adapt to the loss of the placental circuit (clamping of the cord) and initial functioning of the lungs as oxygenators. Pulmonary vascular resistances (PVR) lower, the ductus arteriosus and the foramen ovale close. This unstable period lasts from a few hours to several weeks: it’s the transitional circulation (Figure 14.4), a labile state during which a return to foetal type circulation occurs under the effect of hypoxia, hypothermia, acidosis or stress (Table 14.2).
Figure 14.4: Diagram of transitional circulation with momentary opening of right-to-left shunts by the foramen ovale (FO) and by the ductus arteriosus (DA) following an increase of pulmonary vascular resistances (PVR). The child becomes cyanotic. The direction of flow through the ductus arteriosus depends on the ratio of pressure between the aorta and pulmonary artery; in severe pulmonary hypertensive reactions, it is L→ R in systole, but R → L in diastole.
Transitional circulation presents a series of characteristics [5,7].
- The functional occlusion of the DA occurs between 10 and 15 hours of life, but its anatomical closure takes 4-6 weeks. During this period, a weak left → right (L-to-R) flow continues. An elevation of the PaO2 increases vasoconstriction of the ductus, while a decrease of the PaO2 reopens it. This is true for a child born in due time, because the immature DA does not contract, even in the case of hyperoxia. The prostaglandin E1 releases the musculature of the canal and keeps it permeable, with low or high PaO2; this phenomenon ensures a vicariance by the DA from the aorta in case of low pulmonary flow or from the pulmonary artery in the case of interruption of the aortic arch. Indomethacin, a prostaglandins inhibitor, can induce the closure of the DA in the neonatal period in premature babies. Paracetamol (acetaminophen) also seems to be promising, with less adverse effects [2]. The presence of one of the factors mentioned in Table 14.2 may extend its permeability, leading to a shunt whose direction depends on the ratio between systemic and pulmonary pressures: It may be L-to-R in case of an obstruction to the pulmonary flow (pulmonary or tricuspid atresia) or R-to-L if the obstruction concerns aortic flow (aortic hypoplasia, coarctation).
- The FO closes as soon as the pressure of the LA exceeds that of the RA in the first few days of life. However, anatomical occlusion is not constant: The FO remains permeable in adulthood in approximately 25% of adults [1,8]. Everything that increases PVR (Table 14.3) can cause a L-to-R shunt by this orifice. Even in the absence of pulmonary hypertension, coughing or a Valsalva manoeuvre can raise the pressure of the RA sufficiently to cause a L-R shunt, thus explaining the occurrence of paradoxical embolisms.
- Pulmonary vascular resistances (PVR) are high in utero, but decrease rapidly at birth; they are halved compared to the systemic values after 24 hours of life and then gradually decrease over 5-6 weeks; they reach their adult value around the age of 3 years (Figure 14.5) [3]. The pulmonary vascular bed is particularly responsive during this period; a pulmonary hypertensive (PHT) crisis occurs easily in the event of hypoxemia, hypercarbia, acidosis or a bronchospasm. In the first few days of life, this may lead to a R-to-L shunt by the ductus arteriosus or the foramen ovale (see Figure 14.4). The rapid fall of PVR during the first few hours of life is due to active vasodilation in response to the elevation of the pO2 and the expansion of the newly-ventilated alveoli. By contrast, the transformations of the first few years are related to anatomical changes (arborisation of the pulmonary vascular bed), which can be slowed down or suppressed by the factors mentioned in the Table 14.3. A volume and pressure overload by a L-to-R shunt hinders the decline of PVR and causes a secondary increase in pulmonary arterial pressure.
- The artery and the umbilical vein close shortly after birth, as well as the ductus venosus.
- The mass of the two ventricles is almost equal at birth. The thickness of the ventricle connected to the low resistance lung network (normally the RV) decreases rapidly, while the ventricle connected to the systemic vascular resistances (SVR) thickens.
Figure 14.5: Changes of pulmonary vascular resistances (PVR) after birth (in mmHg/mL/min/kg) [according to ref 3].
The neonatal period is characterised by an increase in pulmonary resistances and reactivity. Very developed pulmonary vascular musculature and low arborisation of vessels induce pulmonary hypertension crises in the case of nociceptive stimulation (Table 14.3). Children suffering from congenital heart defects with a strong increase in pulmonary flow (L-to-R shunt) can retain very muscularised arterioles and permanently high pulmonary resistances. In addition, they are likely to develop vasospastic paroxysmal pulmonary crises, until the pulmonary and systemic pressures ratio is reversed. These crises induce an acute failure of the right ventricle (high PVR) and a systemic insufficiency by low flow (low PVR). They can be triggered by any phenomena which increase the PVR and can occur without warning signs. They are particularly frequent after interventions under cardiopulmonary bypass (CPB), because many humoral, endothelial and mechanical factors related to the latter contribute to the elevation of the pulmonary resistances. A history of sudden changes to the PAP is a good sign of postoperative right ventricular failure.
Adult circulation
Adult circulation ensures a complete separation of saturated arterial and desaturated venous blood, with systemic circulation and pulmonary circulation in series (Figure 14.6).
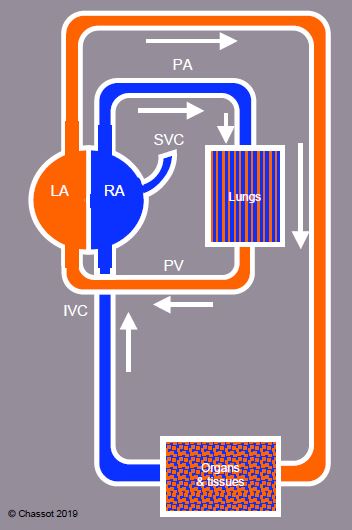
Figure 14.6: Adult circulation ensures a complete separation of saturated arterial and desaturated venous blood, with serial systemic circulation and pulmonary circulation in series.
Fetal and transitional circulation |
Foetal circulation: oxygenated blood from the placenta (SO2 75%) → umbilical vein → liver (ductus venosus) → IVC → RA → foramen ovale → LA → LV → coronary arteries, brain, upper limbs. Desaturated blood of SVC (SO2 40%) → RV → AP → ductus arteriosus → viscera, lower limbs.
Transitional circulation: unstable period during which the ductus venosus (1-2 hours), the ductus arteriosus (< 24 hours) and the foramen ovale (25% remains permeable) close. Hypoxia, acidosis, hypothermia or stress increase the PAP and induce a return to fetal circulation. |
© BETTEX D, BOEGLI Y, CHASSOT PG, June 2008, last update February 2020
References
- AGOUSTIDES JG, WEISS SJ, WEINER J, et al. Diagnosis of patent foramen ovale with multiplane transesophageal echocardiography in adult cardiac surgical patients. J Cardiothorac Vasc Anesth 2004; 18:725-30
- BARDANZELLU F, NERONI P, DESSI A, et al. Paracetamol in patent ductus arteriosus treatment: efficacious and safe? Biomed Res Int 2017; 2017:1438038
- CLARKE WR. Anesthetic care of the infant with abnormal transitional circulation. Problems in Anesthesia 1988; 2:477-89
- DUPUIS C, KACHANER J, FREEDOM R, PAYOT M, DAVIGNON A. Cardiologie pédiatrique, 2nd edition. Paris: Flammarion, 1991, 115-123
- FINEMAN JR, SOIFER SJ, HEYMANN MA. Regulation of pulmonary vascular tone in the perinatal period. Annu Rev Physiol 1995; 57:115-34
- KLOESEL B, DINARDO JA, BODY SC. Cardiac embryology and molecular mechanisms of congenital heart disease - A primer for anesthesiologists. Anesth Analg 2016; 123:551-69
- KUSSMAN BD, HOLZMAN RS. Cardiac embryology: Understanding congenital heart disease for the noncardiac anesthesiologist. Sem Cardiothorac Vasc Anesth 2001; 5: 2-20
- SCHNEIDER B, ZIENKIEWICZ T, JANSEN V, et al. Diagnosis of patent foramen ovale by transesophageal echocardiography and correlation with autopsy findings. Am J Cardiol 1996; 77:1202-9
14. Anesthesia for paediatric heart surgery
- 14.1 Introduction
- 14.2 Pathophysiology
- 14.3 Haemodynamic strategies
- 14.3.1 Classification
- 14.3.2 Left-to-right shunt and high pulmonary blood flow
- 14.3.3 Pulmonary hypertension in children
- 14.3.4 Cyanotic right → left shunt and reduced pulmonary blood flow
- 14.3.5 Cyanotic right → left shunt and reduced systemic blood flow
- 14.3.6 Bidirectional cyanotic shunt
- 14.3.7 Heart diseases without shunting: obstructions and valvular heart diseases
- 14.3.8 Treatment options for neonates
- 14.3.9 Drug therapy
- 14.4 Anaesthetic technique
- 14.5 CPB in children
- 14.6 Anaesthesia for specific pathologies
- 14.6.1 Introduction
- 14.6.2 Anatomical landmarks
- 14.6.3 Anomalous venous returns
- 14.6.4 Atrial septal defects (ASDs)
- 14.6.5 Atrioventricular canal (AVC) defects
- 14.6.6 Ebstein anomaly
- 14.6.7 Anomalies of the atrioventricular valves
- 14.6.8 Ventricular septal defects (VSDs)
- 14.6.9 Ventricular hypoplasia
- 14.6.10 Tetralogy of Fallot
- 14.6.11 Double outlet right ventricle (DORV)
- 14.6.12 Pulmonary atresia
- 14.6.13 Anomalies of the left ventricular outflow
- 14.6.14 Transposition of the great arteries (TGA)
- 14.6.15 Truncus arteriosus
- 14.6.16 Coarctation of the aorta
- 14.6.17 Arterial abnormalities
- 14.6.18 Heart transplantation
- 14.7 Conclusions